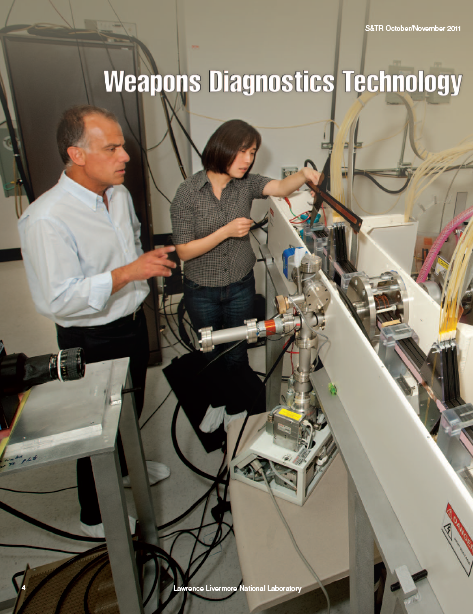
At Compact Particle Acceleration Corporation (CPAC) in Livermore, California, a proton therapy system based on Livermore technologies is being developed to treat cancer patients. Anthony Zografos (left), general manager of CPAC, and Yoko Parker (right) stand alongside the dielectric wall accelerator prototype system. A radio-frequency quadrupole and proton source are to the right of the accelerator. In contrast, existing proton treatment centers are about the size of a basketball arena.
IN 2014, a particle accelerator born out of the Laboratory’s nuclear weapons program may begin to appear in hospitals to deliver lifesaving proton therapy to cancer patients who would otherwise receive traditional and often dangerous radiation treatments. The path to this success has been long and challenging, but the payoff for patients is enormous.
Scientists recognized the benefits of radiation as a method for treating cancer in the late 1800s, even before x rays were used as an imaging device. A medical student, Emil Grubbe, noted that his hands peeled when exposed to x rays and recognized x rays as a possible way to remove damaging tissue. Grubbe’s use of
x rays to treat a woman with advanced breast cancer slowed the tumor’s growth. Today, radiation therapy can be a highly successful method of treatment, but it also often damages healthy tissue around the tumor as the radiation beam passes through the body. Ever more sophisticated methods of delivering radiation have reduced collateral damage, but it is still virtually impossible to avoid.
Enter proton therapy, whose properties are entirely different from x- or gamma-ray treatments. Because of their relatively large mass, protons have minimal lateral side scatter in the tissue. The proton beam is highly focused on the tumor and creates only low-dose side effects in surrounding tissue. A proton beam of a given energy has a certain range, allowing only very few protons to penetrate beyond that distance. Calibrating the energy of the beam to the depth of the tumor delivers the appropriate proton dose specifically to the tumor. Tissue closer to the surface of the body, above the tumor, receives less radiation and therefore less damage, while tissue around the tumor receives almost no protons. Proton therapy is considered especially effective for the treatment of eye cancer and for children who require radiation. It is also gaining ground as a method for treating prostate cancer because damage does not occur in the surrounding nerves that are important for maintaining sexual function.
Despite the effectiveness of proton therapy, only 31 proton treatment centers exist in the world, with just nine in the U.S. The current delivery method requires what is essentially a giant bunker to house a cyclotron or synchrotron and patient treatment rooms. The beamline, weighing several hundred tons, is surrounded by concrete walls 3 meters thick to shield patients and operators from the heavy dose of neutrons that are a side effect of beam loss in such systems. Three-meter-thick walls must also surround each therapy room, which are typically three stories high to accommodate the gantry vault that the patient lies in. Given that a proton treatment center is about the size of a basketball arena and costs more than
$100 million, it comes as no surprise
that few hospitals can afford one.
The proton therapy system based on Laboratory technologies and being refined by Compact Particle Acceleration Corporation (CPAC) of Livermore, California, will revolutionize proton delivery. The 4-meter-long linear accelerator will use intensity-modulated proton therapy to treat the patient and will be available for a small fraction of the cost of today’s systems. The system will deliver protons more directly to the patient than do typical scattered proton beams and may require no more shielding than an ordinary x-ray facility. Given the projected low cost of the system and proven effectiveness of protons for the treatment of many cancers, hospitals will be more likely to retrofit one or more of their traditional radiation facilities to deliver protons instead.
In June, when CPAC completed the construction of its first precommercial prototype system, the company had taken a major step toward providing the world’s most precise and compact proton accelerator for treating cancer and other solid tumors. Anthony Zografos, CPAC general manager, says, “The Laboratory has been an excellent partner for us. Livermore researchers, who are extremely knowledgeable about the technology, have assisted us in our efforts to improve the system. This CRADA [Cooperative Research and Development Agreement] has been a great success.”
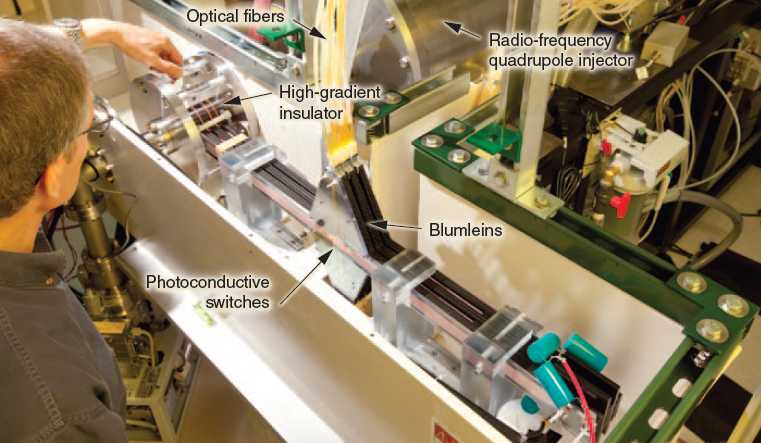
Livermore physicist George Caporaso works on the dielectric wall accelerator assembly at CPAC. The metal cylinder (top right) contains the radio-frequency quadrupole injector.
Present at the Creation
The idea for the compact proton accelerator came from a team, led by physicist George Caporaso, in Livermore’s Beam Research Program in the Physical and Life Sciences Directorate. The program’s responsibilities include the development of advanced accelerators and technologies. The novel design came about because of the potential benefits of performing x radiography with a small electron accelerator to augment the radiographic capabilities of Livermore’s Flash X Ray (FXR) and Los Alamos National Laboratory’s Dual-Axis Radiographic Hydrodynamic Test (DARHT) facilities.
FXR and DARHT are enormous machines, tens of meters long, and use a Livermore-developed linear induction accelerator to take x-ray images of very dense, nonnuclear test devices as they implode. Both systems are essential tools of the National Nuclear Security Administration’s Stockpile Stewardship Program, which, in the absence of underground nuclear testing, must ensure that the nation’s existing warheads remain safe, secure, and reliable well into the future.
An induction accelerator comprises a series of electrically independent modules, each pulsed in turn to push energetic particles forward, increasing their energy with every pulse. FXR and DARHT are long because they need to deliver photons at very high energies and because their acceleration gradient, the rate of energy increase over distance, is low, less than 1 megaelectronvolt (MeV) per meter. A method was needed to vastly increase the acceleration gradient and deliver equivalent energies across a distance of just a few meters.
The solution was a high-gradient insulator (HGI), a “sandwich” made from very thin layers of insulating and conducting materials across which large electric fields are applied to accelerate charged particles. HGI, which won an R&D 100 Award in 1997, forms the beam tube of a dielectric wall accelerator (DWA). “HGI was invented by an engineer at Sandia [National Laboratories] and was brought to our attention by Steve Sampayan, an engineer in our group who had worked at Sandia,” says Caporaso. But Sampayan and his team took the invention to new lengths, so to speak. Livermore’s HGI is constructed from conducting layers made of metal, such as stainless steel, alternating with insulating layers of plastic, such as polystyrene.
The small-scale induction accelerator comprises a stack of HGIs that can hold extreme voltages. A particle injector starts the action, and transmission lines made of dielectric materials and embedded conductors produce the electric field that propels the charged particles forward in the tube. The transmission lines are called Blumleins, after a technology developed by the prolific British inventor Alan Blumlein, who also developed stereophonic sound. A laser delivers power to switches in the Blumleins through an optical-fiber distribution system. The tiny, solid-state silicon carbide optical switches on the Blumleins open and close at high speeds to control the high-power voltage that fires each Blumlein, thereby increasing the energy of the particles as they traverse the tube.
With the opening and closing of each switch, one section at a time of DWA is energized, creating a “virtual traveling wave” that pushes the energized particles down the tube. Caporaso likens the phenomena to “the wave” that sports fans perform around a stadium. The ones standing are pushing the particles. They sit down and their neighbors continue the push. One thousand Blumleins may be fitted around a 2-meter tube, each one fired on command to control the speed, form, amplitude, and length of the beam pulse.
“If the whole tube were energized at once,” says Caporaso, “it would short out. But that problem is solved by energizing only a very small area of the tube at a time.” This method also places less stress on materials in the device.
The DWA components were integrated into an accelerating module for radiography, but a complete accelerator was not built because the weapons application was not pursued. However, at about that time, fellow physicist Dennis Matthews asked Caporaso if DWA could deliver protons instead of electrons. Matthews was leading a team developing biomedical devices at the Laboratory and also working at the newly established University of California (UC) Davis Cancer Center, in which Livermore was an active collaborator. Matthews knew that Ralph deVere White, a urological oncology specialist and director of the center, was on the hunt for a more compact method for proton therapy.
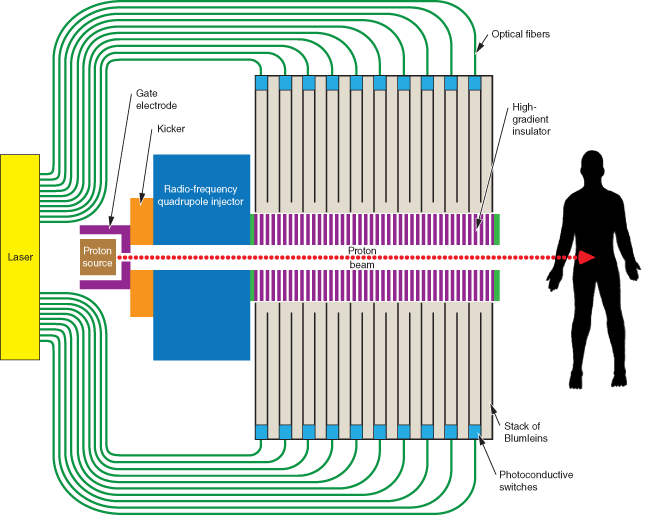
This schematic illustrates the flow of protons from a source through the dielectric wall accelerator assembly to a patient. A commercially available ion source sends protons into a “kicker” that injects pulses of protons into a radio-frequency quadrupole, which compresses the protons into short bunches. Switches along the accelerator open and close at high speeds to control the voltage and increase the energy of particles zooming down the accelerator. Careful control of the switching mechanisms creates a beam pulse with the speed, form, amplitude, and length needed for a particular patient.
Compact Accelerator Comes to Life
Both Matthews and deVere White were excited about the prospects for Livermore’s novel electron delivery methodology. However, much work remained for DWA to be modified for use as a proton delivery medical device. The components developed for radiography had been designed to deliver electrons in long pulses at approximately 10 million volts per meter. But effective proton therapy required very short pulses and a gradient of at least 40 million volts per meter.
In 2004, the Laboratory Directed Research and Development Program funded a project to determine the feasibility of DWA technology for medical use. Later, UC Davis invested millions of dollars, confident that the DWA technology would be the effective, compact proton therapy device so needed by the oncology community. Matthews notes that the goal of this project was to prove the technology’s value and make it attractive to investors, without whom DWA for proton therapy would never get beyond the Laboratory gates.
It proved to be attractive enough for TomoTherapy, Inc., a company headquartered in Madison, Wisconsin, and already active in the development and sale of traditional radiation treatment systems. In a 2007 technology transfer agreement, TomoTherapy entered the proton therapy business with funding for the continued development of the new accelerator. Several TomoTherapy researchers joined the Laboratory team. With TomoTherapy’s financial and personnel backing, Livermore was able to develop and successfully test a prototype in December 2007, of which the DWA portion was about 2 centimeters long. According to Livermore engineer Jim Watson, who has been a member of the DWA team since 2007, this test proved the world’s first compact DWA accelerator, with particle injector and diagnostics. By December 2008, the team had demonstrated that the device could accelerate protons.
DeVere White notes that this technology is unique for proton therapy. No other company is close to delivering a device with the small size, low cost, and treatment effectiveness of DWA. Says deVere White, “Some cancer experts wonder whether the $150 million price tag is worth the advantages that proton therapy can provide. This solution takes the cost issue away.”
Ralph deVere White, director of the University of California (UC) Davis Cancer Center, was instrumental in UC Davis partnering with the Laboratory to pursue a compact proton therapy device needed by the oncology community.
(Courtesy UC Davis Cancer Center.)
CPAC Enters the Picture
In 2008, TomoTherapy announced its participation in the new venture CPAC, located just blocks from the Laboratory in Livermore. In 2009, Zografos, who has considerable medical technology experience, was hired and began to build his staff of engineers and other experts to refine the transferred technology. Today, CPAC has 10 employees. “In addition, a number of Laboratory staff are in and out almost constantly assisting us,” says Zografos. Teams of experts on a technical advisory board and a clinical advisory board also support CPAC. Rock Mackie, cofounder of TomoTherapy, is chair of the clinical advisory board.
Zografos notes that except for the Livermore-designed accelerator, all components are commercially available and almost all are from manufacturers in California. “Our entire effort here at CPAC is on the accelerator,” he says. “The development of the rest of the system, including the laser, high-voltage equipment, and fiber optics, is outsourced.” One decision made early on was whether to use Livermore’s particle injector or a commercial radio-frequency quadrupole (RFQ). The prices were the same, but the technical advisory board chose the commercial product.
The first system test in March included a pulser composed of a commercial ion source and a 2-MeV RFQ that serves as an injector for delivering subnanosecond protons into DWA. Both pulser components are conventional, proven technologies. In addition, a novel 1-centimeter “kicker” between the ion source and RFQ acts as a gate to inject a single pulse of protons into the quadrupole. RFQ then compresses these protons into a bunch about 200 picoseconds long when exiting. Physicist Gary Guethlein led a small team to develop the compact kicker. Guethlein and physicist Steve Falabella also created a timing system built of commercially available components to synchronize RFQ with the kicker and DWA switching laser. Protons are thus in DWA only as needed and with almost no jitter.
As development of the accelerator progresses, Caporaso continues to lead Livermore’s work. Guethlein visits CPAC often to assist with testing and design. Another frequent visitor is physicist Yu-Jiuan Chen, who is responsible for optimizing the delivery of protons. As such, she plays a major role in specifying design requirements for the Blumleins, the high-gradient insulator, the optical switch, and other parts of the accelerator. If the commercial components are not ideal, her task is to combine them into a workable design.
Chen notes that while the switch design is final, she, Brian Poole, and others are still working on the Blumleins, combining tests at CPAC with computer simulations at Livermore. The Blumlein’s structure is critical because it determines the form, length, and amplitude of the pulse. “Currently, each Blumlein is 2 millimeters thick,” she says. “Thinner is better to maximize the acceleration gradient. A thicker Blumlein dilutes the gradient.”
To test the insulator, CPAC has installed a high-voltage pulser in an electromagnetically shielded chamber at its facility. Various configurations, materials, and fabrication methods for the insulator are examined to determine the combination that can best withstand the high gradients. Livermore and CPAC staff work together on the tests, and Chen’s team performs simulations that aid experimentation. The Laboratory’s Watson is often at CPAC as well, helping with testing of HGI and other engineering functions.
Chen says, “Little room exists for external focusing lenses in the short length of the accelerator, which would help keep the beam’s ‘spot size’ small and tightly focused.” Dealing with this challenge requires such tasks as developing focusing methods that provide simultaneous longitudinal and transverse stability, flattening the accelerator waveform to reduce radial defocusing, and maintaining beam quality. The phase of the first Blumlein firing has to match that of the proton source, and the phase velocity of Blumlein firings must match the proton velocity throughout the accelerator. Thanks to the kicker and timing system, those parts of the accelerator are in synch. Diagnostic devices designed by Guethlein and Falabella are installed along the length of DWA about every meter to ensure beam quality. Above all, says Chen, “We have to produce a practical design.”
“The first prototype, tested this spring with a DWA of about 5 centimeters, proved our ability to accelerate protons,” says Zografos. With subsequent tests, more modules will be added, lengthening the accelerator.
The accelerator for the second prototype will be about 20 centimeters long. That unit, scheduled for testing in the first quarter of 2012, will prove the clinical viability of the device. This prototype will not be used for treatment but rather to demonstrate that adding more modules does indeed increase output energy and that the machine can be scaled up to the energy levels needed to deliver therapeutic protons. According to Livermore simulations, a 4-meter accelerator would deliver 160 MeV with clinical precision. A shorter, 2-meter accelerator would deliver 120 MeV.
The third and final prototype, scheduled for testing in 2013, will combine both technical and clinical viability in an integrated system that could be installed in hospitals. Says Zografos, “We have to know whether the device can be reasonably priced and what the cost of ownership will be in terms of maintenance, spare parts, and so on.”
Accuray, Inc., which acquired TomoTherapy in June, will likely handle final integration of all the system components. Clinical testing may take place at the University of Wisconsin at Madison’s hospital, although the testing location has yet to be finalized. Approval for patient use by the Food and Drug Administration will be relatively simple because proton therapy already has the agency’s approval. This particular system need only prove that it can deliver protons at therapeutic levels.
Accuray–TomoTherapy will also likely be responsible for the manufacture, sale, and distribution of this lifesaving device to hospitals. To facilitate a hospital’s transition from x- and gamma-ray treatment to proton therapy, CPAC will deliver a fully integrated product that allows a hospital to easily begin implementing proton therapy. The system will incorporate treatment planning, quality assurance software and tools, volumetric computed tomography imaging, beam delivery, and patient positioning.
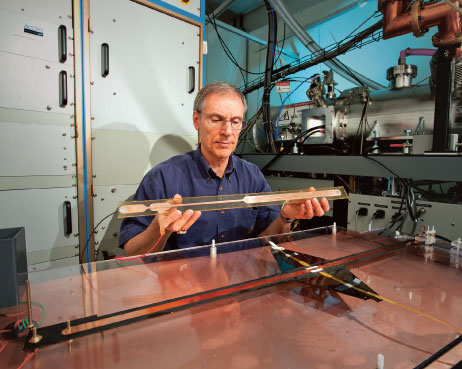
Caporaso examines CPAC’s newest Blumlein design. Tests at CPAC are combined with computer simulations at Livermore to produce a practical design.
Lasting Relationships
CPAC holds the exclusive commercial rights to more than 30 DWA-related patents or patent applications and has the license for nonmedical uses for the optical switches and DWA. “The technology could be used for imaging and cargo inspection, for example,” says Zografos. Both are high on the Department of Energy’s list of important national security projects, so Livermore involvement will surely continue.
DeVere White observes that the Laboratory’s application of a stockpile stewardship technology to the medical field is a highly effective use of tax dollars. “It leverages monies spent on national security without diverting the Laboratory from its primary mission,” says deVere White. “Without Livermore, the Cancer Center, TomoTherapy, and CPAC, this technology for cancer treatment would not exist. Cancer patients will be the beneficiaries of an exceptional partnership.”
—Katie Walter
Key Words: Accuray, Inc.; cancer treatment; Compact Particle Acceleration Corporation (CPAC); Cooperative Research and Development Agreement (CRADA); dielectric wall accelerator (DWA); high-gradient insulator (HGI); proton therapy; x-radiation therapy; technology transfer; TomoTherapy, Inc.
For further information contact George Caporaso (925) 422-7852 (caporaso1@llnl.gov).
©